Abstract
Objectives. Myeloperoxidase (MPO) has been implicated in Parkinson´s disease (PD). The objective was to look at the relationship of MPO concentration in serum and cerebrospinal fluid (CSF) with clinical variables of PD.
Methods. In this prospective, observational, and cross-sectional study, MPO concentration in serum and the CSF was analyzed in 36 patients with idiopathic PD and 30 controls, who were enrolled from 2012 to 2017. In the group of patients, correlation of MPO content with demographic, clinical and tomographic variables was examined. The extent and degree of nigrostriatal dopaminergic cell loss was evaluated by using SPECT and 123I-Ioflupane, radioligand that has binding affinity for dopamine-transporter (DAT).
Results. Serum MPO concentration, not CSF MPO content, was significantly higher in the patients (p<.0001). Significant correlation values were found between serum MPO concentration and rating scales of motor severity (Hoehn-Yahr, MDS-UPDRS part-III), and percentage reduction of DAT binding on basal ganglia (p<.0001). Patients with moderate-advanced disease (Hoehn-Yahr stage 3) showed significantly higher serum MPO content relative to patients with early disease (Hoehn-Yahr stages 1-2, p<.0001). DAT binding was reduced on all striatal regions, and signal reduction was higher in the putamen relative to the caudate nucleus (p<.0001). Percentage reduction of DAT binding on the striatum and putamen significantly correlated with rating scales of motor severity.
Conclusions. Myeloperoxidase content in serum is increased in PD patients and correlates with motor severity degree and loss of dopamine-transporter binding on basal ganglia. The results allow proposing that the measurement of MPO level in serum could be useful for PD diagnosis, and that the inhibition of serum MPO would be a promising therapeutic tool. The study also confirms that the putamen shows higher reduction of DAT binding than the caudate nucleus, and degree of nigrostriatal dopaminergic cell loss is well quantified with rating scales of motor severity.Resumen
Objetivos. La mieloperoxidasa (MPO) se ha relacionado con la enfermedad de Parkinson (EP). El objetivo fue analizar la relación entre la concentración de MPO en suero y líquido cefalorraquídeo (LCR) con variables clínicas de la enfermedad.
Métodos. En este estudio prospectivo, observacional y transversal, la concentración de MPO en suero y LCR se analizó en 36 pacientes con EP idiopática y 30 controles, reclutados de 2012 a 2017. En el grupo de pacientes, se evaluó la correlación entre la concentración de MPO y parámetros demográficos, clínicos y tomográficos. El grado de pérdida dopaminérgica se cuantificó mediante SPECT y la unión de I123-Ioflupano al transportador de dopamina (DAT).
Resultados. La concentración de MPO sérica, no en LCR, fue mayor en los pacientes (p<0,0001), y se correlacionó significativamente con escalas de gravedad motora (Hoehn-Yahr, MDS-UPDRS III), y el porcentaje de reducción de la señal de unión DAT (p<0,0001). Los pacientes con enfermedad moderada-avanzada (grado 3 de Hoehn-Yahr) presentaban mayores niveles séricos de MPO que los pacientes con EP temprana (grados 1-2, p<0,0001). La señal de unión DAT se redujo en todas las regiones estriatales, y dicha reducción fue mayor en putamen que en núcleo caudado (p<0.0001). La reducción porcentual de la señal dopaminérgica en estriado y putamen se correlacionó con las escalas de gravedad motora.
Conclusiones. Se demuestra que la mieloperoxidasa sérica está elevada en la EP y se relaciona con la gravedad motora y la reducción de señal dopaminérgica estriatal. Los resultados permiten proponer que cuantificar los niveles de MPO en suero podría ser útil para el diagnóstico de la EP, y que la inhibición de dicha enzima podría ser un arma terapéutica. El estudio confirma que el putamen presenta mayor pérdida de señal dopaminérgica que el núcleo caudado, y las escalas de gravedad motora reflejan adecuadamente la pérdida dopaminérgica nigroestriatal.Keywords: Parkinson’s disease; Myeloperoxidase; Cerebrospinal fluid; Serum; Dopamine-transporter; Basal ganglia.
Palabras clave: Enfermedad de Parkinson; Mieloperoxidasa; Suero; Líquido cefalorraquídeo; Transportador de dopamina; Ganglios basales.
1. Introduction
The enzyme myeloperoxidase (MPO) has been implicated in the development of Parkinson´s disease (PD) (1-5). Thus, MPO is expressed by neurons in the substantia nigra pars compacta, and this enzyme contributes to α-synuclein pathology (6, 7). Studies on MPO cell expression in PD have yielded conflicting results. Some authors have reported increased MPO expression in surviving neurons and active glial cells in the substantia nigra in diseased patients and animal models of PD (7-9). Other authors have described that the number of MPO-immunoreactive blood-derived cells, rather than microglia, is increase in basal ganglia of human PD brains (10).
MPO represents an essential component of the immune defense system and is expressed by peripheral phagocytes (neutrophils and monocytes/macrophages) and brain glial cells (microglia and astrocytes) (3-5, 11). The main biochemical function of MPO is to catalyze the reaction between hydrogen peroxide and chloride giving rise to hypochlorite, chlorinated products, and other reactive species, all powerful microbicidal molecules (5, 12-14). It is worth noting that inflammation and oxidative stress are implicated in PD pathogenesis (15-17), and MPO-derived products are pro-inflammatory and pro-oxidant compounds which can damage neurons (2-6, 18-20).
The cytotoxicity of MPO and its localization in brain cells indicate its clinical importance, and this enzyme has been proposed to be a target for antiparkinsonian treatment (10, 22, 23). Recently, Jucaite et al (23) have provided support for proof of mechanisms of AZD3241, selective inhibitor of MPO, in PD patients, though the authors recognize that longer treatment is required to know if the effects are beneficial for therapy. To date, no study has examined how MPO in human biofluids relates to motor features in idiopathic PD. The hypothesis is that myeloperoxidase in blood serum or the cerebrospinal fluid (CSF) might be involved in dopaminergic cell loss in PD. The objective was hence to look at the relationship of MPO concentration in serum and the CSF with demographic, clinical and tomographic variables of the disease. The extent and degree of nigrostriatal dopaminergic cell loss was evaluated by using single-photon emission computed tomography (SPECT).
2. Material and methods
2.1. Participants
This is a prospective, observational, and case-control study, where thirty-six patients with idiopathic PD and 30 controls were enrolled at Hospitals Macarena and Valme, Sevilla, Spain, from 2012 to 2017. Patients were diagnosed of PD if they present all three classic motor signs of parkinsonism (bradykinesia, rigidity, and resting tremor), and a reliable loss of dopaminergic signal on basal ganglia, as measured with 123I-Ioflupane DAT-SPECT (24-26). To exclude hereditary forms of Parkinsonism, those patients with family members with PD, younger than 45 years old, or with atypical deficits, were discarded. Patients at advanced stages of PD (Hoehn-Yahr stages 4 and 5) were not included in the study. Control subjects, free of neurological disorder, were recruited from volunteers or patients´ relatives. They were group-matched by age and gender to PD subjects. Controls were excluded if they had a first-degree family member with a neurological disorder. Informed consent forms were obtained from all the subjects.
All protocols were approved by the internal ethics and scientific boards of Junta de Andalucia (PEIBA, CEI-Sevilla-Sur #5/10/2015 & #2017-121418738), Hospital Valme (CEI-Valme, #10/05/2018 & #1694-M1-19), Hospital Macarena (CEI-Macarena, 19/05/2010 & 29/10/2013), and Universidad de Sevilla (CEI-27/05/2010). The subjects’ consent was obtained according to the Declaration of Helsinki (BMJ 1991; 302:1194).
2.2. Demographic and clinical information
A standardized study was carried out, including information such as age, gender, hypertension, diabetes, rating scales of disease severity, and pharmacological treatment. Hypertension was diagnosed when blood pressure repeatedly (two measures fifteen days apart) exceeded 140 mmHg (systolic) and/or 90 mmHg (diastolic) or when a subject was taking antihypertensive medication. Diabetes was diagnosed according to WHO criteria (fasting plasma glucose ≥ 126mg/dl or 2–h plasma glucose ≥ 200mg/dl). Rating scales were the modified Hoehn-Yahr staging, and the International Parkinson and Movement Disorder Society-Sponsored revision of the Unified Parkinson’s Disease Rating Scale (MDS-UPDRS). Antiparkinsonian medication was expressed as levodopa equivalent dose (LED, mg per day), according to an established formula (27).
Individuals suffering from liver, renal, hematological, and cardiovascular dysfunctions, as well as malabsorption, dementia, autoimmune diseases, acquired immunodeficiency syndrome, cancer, infectious conditions or taking statins or anti-inflammatory drugs were excluded from the study (peroxidase markers may be altered in such conditions). Since alcohol, tobacco and caffeine can also alter oxidative biomarkers in biofluids, all participants were non-alcohol drinkers, non-smokers, and non-coffee drinkers, according to established criteria (28, 29).
2.3. Single-photon emission computed tomography study
SPECT studies were performed according to a standardized protocol. All SPECT scans were performed, quantitatively analyzed, and visually assessed by expert physicians at the Service of Nuclear Medicine. The radioligand 123I-Ioflupane was used for classifying the extent and degree of dopaminergic cell loss. 123I-Ioflupane has a high binding affinity for presynaptic dopamine-transporter or DAT (30-34). Four hours after intravenous injection of 185 MBq of 123I-Ioflupane, scans were acquired on a double-head gamma-camera (Symbia-T2, Siemens) using high-resolution and low energy collimators, 180º, 128 projections, 30 s per view, and a 128 x 128 matrix. Data were reconstructed by filtered backprojection, transferred to a workstation for quantitative analysis (Syngo software). All individual patient studies were co-registered automatically to a mean template derived from studies of healthy volunteers. This template was used to define a 3-dimensional ROI map for the striatum, the caudate nucleus, the putamen, and the occipital cortex (OC) as reference region. Specific binding ratio (SBR) of the radioligand was calculated by substracting the mean counts per pixel in the occipital cortex (OC), from the mean counts per pixel in the basal ganglia nucleus of reference (ROI) and dividing the results by the mean counts per pixel in the OC ([ROI-OC]/OC).
SBR was calculated for the 4 striatal regions (left caudate, right caudate, left putamen, right putamen), as well as the striatum (left striatum, right striatum). The relation between observed SBR versus SBR as expected for individual’s age and gender was calculated. Afterwards, this value was transformed into percentage reduction of SBR (%rSBR) as follows: %rSBR = (100-([observed SBR/expected SBR] x 100)). Expected SBR value was calculated by using the formulas proposed by the European multicenter database of healthy controls for [123I]FP-CIT SPECT (ENC-DAT study, promoted by the European Association of Nuclear Medicine) (33, 34), as follows:
Females:
Expected SBR in the striatum = 7.15 – (0.03 x age in years)
Expected SBR in the caudate nucleus = 7.232 – (0.0273 x age)
Expected SBR in the putamen = 7.116 – (0.0339 x age)
Males:
Expected SBR in the striatum = 6.75 – (0.03 x age)
Expected SBR in the caudate nucleus = 6.8 – (0.02733 x age)
Expected SBR in the putamen = 6.702 – (0.0339 x age)
The minimum putamen (calculated as the minimum SBR value from either the left or right putamen) was determined, and a subject was considered to have a normal DAT binding if minimum putamen SBR was >80% versus SBR as expected for age and gender. A subject was considered to have a deficit in DAT binding if the putamenal SBR was less than 65% as predicted for individual’s age and gender (26). Regarding subjects with percentage reduction of SBR between 65 and 80% relative to normal, visual interpretation was used to determine eligibility, following established criteria (25, 26, 32-34).
2.4. Serum and cerebrospinal fluid collection and ELISA analysis
Myeloperoxidase was measured in blood serum and the cerebrospinal fluid. Blood was obtained by venipuncture. Five ml of blood were collected in gel-coated tubes to obtain serum (BD Vacuotainer, Madrid). Serum was centrifuged at 3000 rpm during 10 min, and then it was frozen at -80ºC in 0.5 ml aliquots. CSF was collected by expert physicians by using lumbar puncture. Two ml of CSF were collected, aliquoted, coded, and rapidly frozen at -80ºC. A 0.5-ml fresh collection was employed to observe the absence of traumatic puncture, and to quantify red cells (CSF with >500 red cells/microL was discarded). Serum and CSF aliquots were unfrozen and sonicated with homogenizing solution (150 mM NaCl, 50 mM HEPES, 1 mM phenylmethylsulfonil-fluoride, 0.6µm leupeptin, 1% Triton X-100, pH 7.4). Myeloperoxidase concentration was evaluated with an Enzyme-linked Immunosorbent Assay kit (Myeloperoxidase Human Instant ELISA™ Kit, BMS2038INST, ThermoFisher Scientific, USA), following manufacturer’s instructions. The sensitivity of the ELISA assay is 26 pg/ml. Each sample was analyzed in duplicate (serum, 1/50 dilution; CSF, undiluted).
2.5. Statistics
Two groups were compared with the Student’s t test (quantitative variables) or the χ2 test (dichotomous variables). Correlations between variables were studied with the Pearson’s test, and values were adjusted for age, gender, and age-at-PD onset. Data normalization was verified with the Shapiro-Wilk test. The patients were classified into subgroups according to the Hoehn-Yahr staging (early disease, stages 1 and 2; moderate-advanced disease, stage 3), and these subgroups were compared. Since many variables were analyzed, the Bonferroni correction was used. Thus, the significance level was set at 0.005 to avoid making Type I errors. Data were analyzed with Minitab19 statistical package (AddLink Software Científico, Spain).
3. RESULTS
3.1. Demographic and clinical parameters, and MPO concentration
First, basic demographic and clinical features in the patients and control participants were compared. Hypertension was found to be significantly more frequent in PD patients than controls (χα2=11.726, p<.001), as shown in Table 1. Serum MPO concentration, not CSF one, was significantly higher in the patients (t=4.9781, p<.0001, Table 1). Serum/CSF ratio of MPO level was found to be significantly higher in PD patients (t=4.5062, p<.0001, Table 1). Individual values of CSF and serum MPO concentration in all participants are shown in Figure 1A.
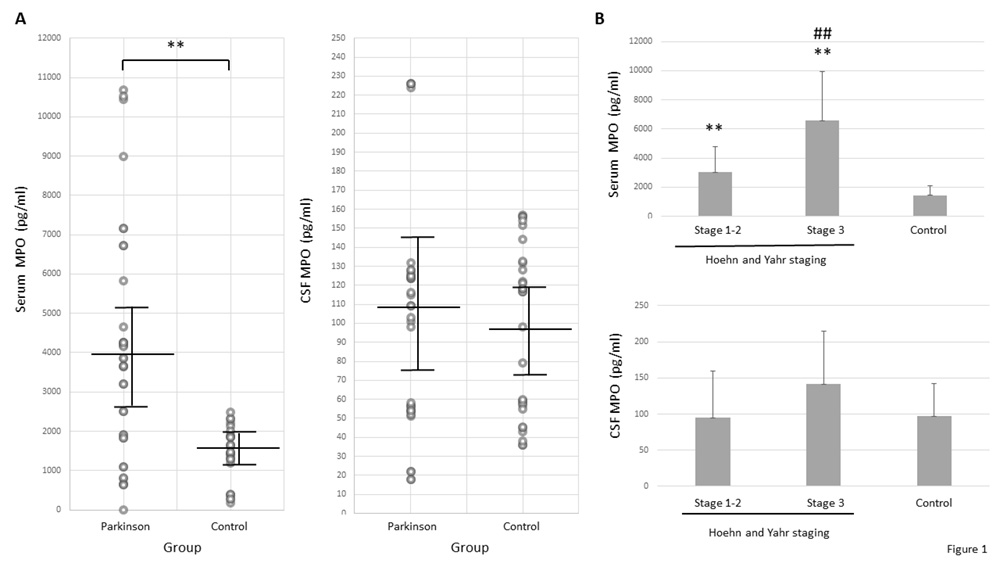
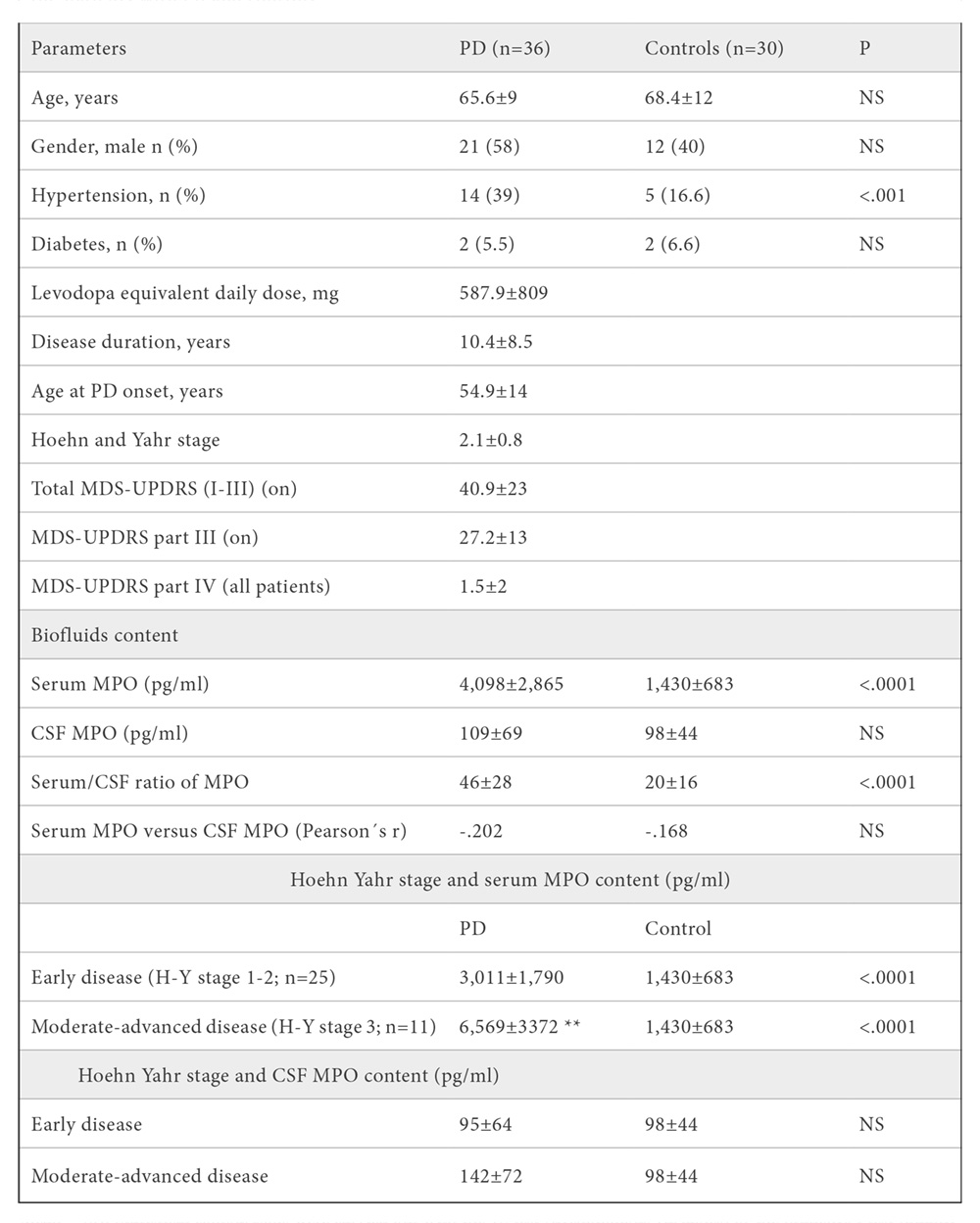
Mean ± SD. Statistical comparisons were carried out with the χ2 test (dychotomous variables) or the Student’s t test (quantitative variables), and Bonferroni correction. ** P=.0002 vs. early disease. Abbrev.: CSF, cerebrospinal fluid; H-Y, Hoehn and Yahr; PD, Parkinson’s disease; MPO, myeloperoxidase; NS, no significant; MDS-UPDRS, International Parkinson and Movement Disorder Society-Sponsored revision of the Unified Parkinson’s Disease Rating Scale; P, two-tailed probability value.
Since hypertension was found to be more frequent in PD patients, the influence of this factor on MPO level was further analyzed. MPO concentration was quantified in patients with or without hypertension, as well as in controls with or without high blood pressure.
MPO concentration was found not to be significantly different in patients with hypertension (3,252±1,836 pg/ml) relative to patients without high blood pressure (4,058±2,082 pg/ml), as well as in control participants with hypertension (1,662±305 pg/ml) versus controls without high blood pressure (1,423±690 pg/ml).
3.2. DAT-SPECT parameters
Regarding DAT-SPECT, all striatal regions (the putamen and the caudate nucleus) showed loss of nigrostriatal dopaminergic neuronal terminals in the cohort of patients, as quantified through percentage reduction of SBR or %rSBR (Table 2). The absolute value of specific binding ratio (SBR) was significantly lower in the left and right putamen versus right and left caudate nucleus, respectively (right, t=7.4626; left, t=9.1306, p<.0001; Table 2). Percentage reduction of SBR (%rSBR) was found to be significantly higher in the left and right putamen relative to the right and left caudate nucleus, respectively (right, t=4.2726; left, t=5.4527, p<.0001; Table 2). Individual values of specific binding ratio of 123I-Ioflupane on basal ganglia, and percent reduction of DAT binding in patients with idiopathic Parkinson’s disease are available upon request.
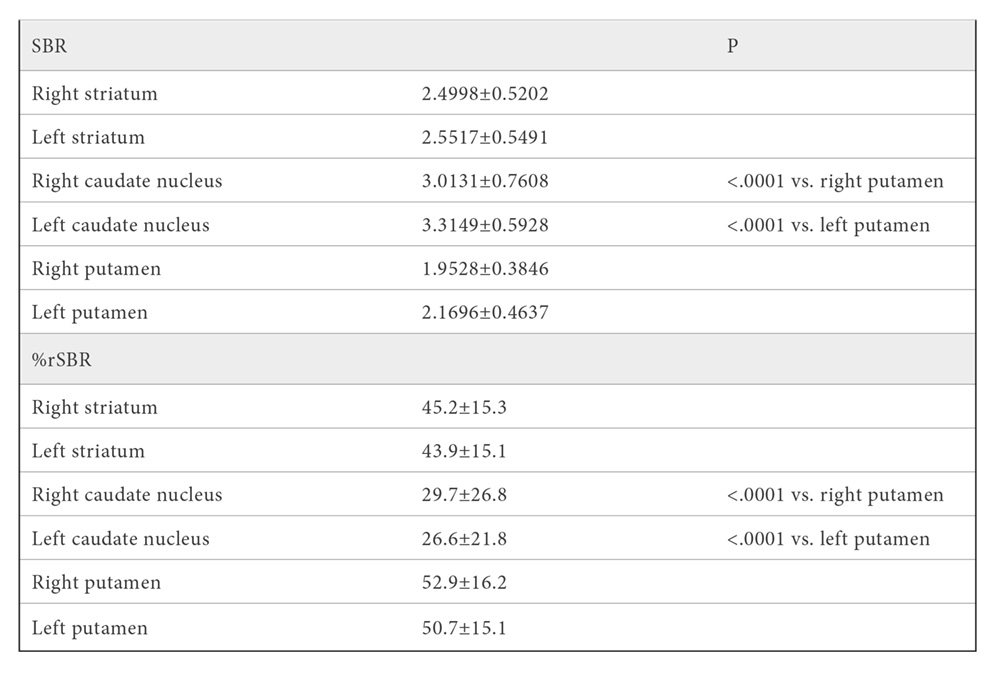
Mean ± SD. Statistical comparisons were carried out with the Student’s t test, and Bonferroni correction of significance. Abbrev.: DAT-SPECT, Dopamine-transporter Single Photon Emission Computed Tomography; P, two-tailed probability value; SBR, specific binding ratio; %rSBR, percentage reduction of SBR.
3.3. Correlation of serum and CSF myeloperoxidase concentration with demographic, clinical and DAT-SPECT parameters
Significant correlations were found between serum MPO concentration and the rating scales Hoehn-Yahr staging (p=.0003) and motor MDS-UPDRS part III (p=.005), as well as percentage reduction of SBR or %rSBR on basal ganglia (p<.001), as shown in Table 3 and Figure 2. PD patients were divided into two subgroups according to disease severity: early disease, Hoehn-Yahr stages 1 and 2, n=25; and moderate-advanced disease, Hoehn-Yahr stage 3, n=11 (no patients at stages 4 or 5). Statistical comparisons revealed that serum MPO concentration was significantly higher in both PD subgroups than in controls (early disease, t=4.496, p<.0001; moderate-advanced disease, t=8.0719, p<.0001), and that patients with moderate-advanced disease showed significantly higher serum MPO content relative to patients with early disease (t=4.1533, p=.0002), as shown in Table 1 and Figure 1B. Taken together, the results indicate that serum MPO content is related to degree of motor severity of PD. Finally, no significant correlation was found between serum MPO concentration and CSF MPO content (patients, r= -.202; controls, r= -.168, NS; Table 1), indicating that both parameters are independent to each other.
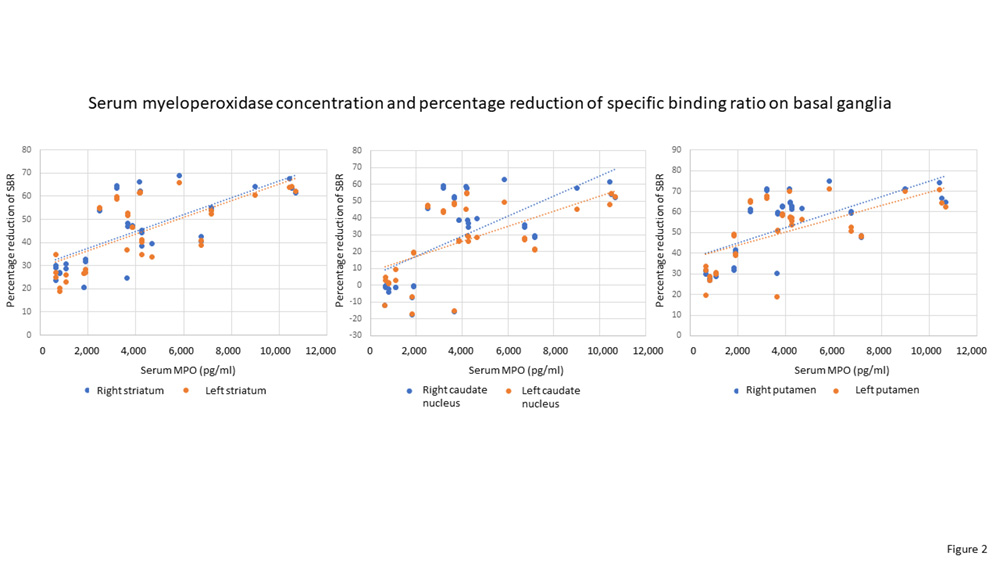
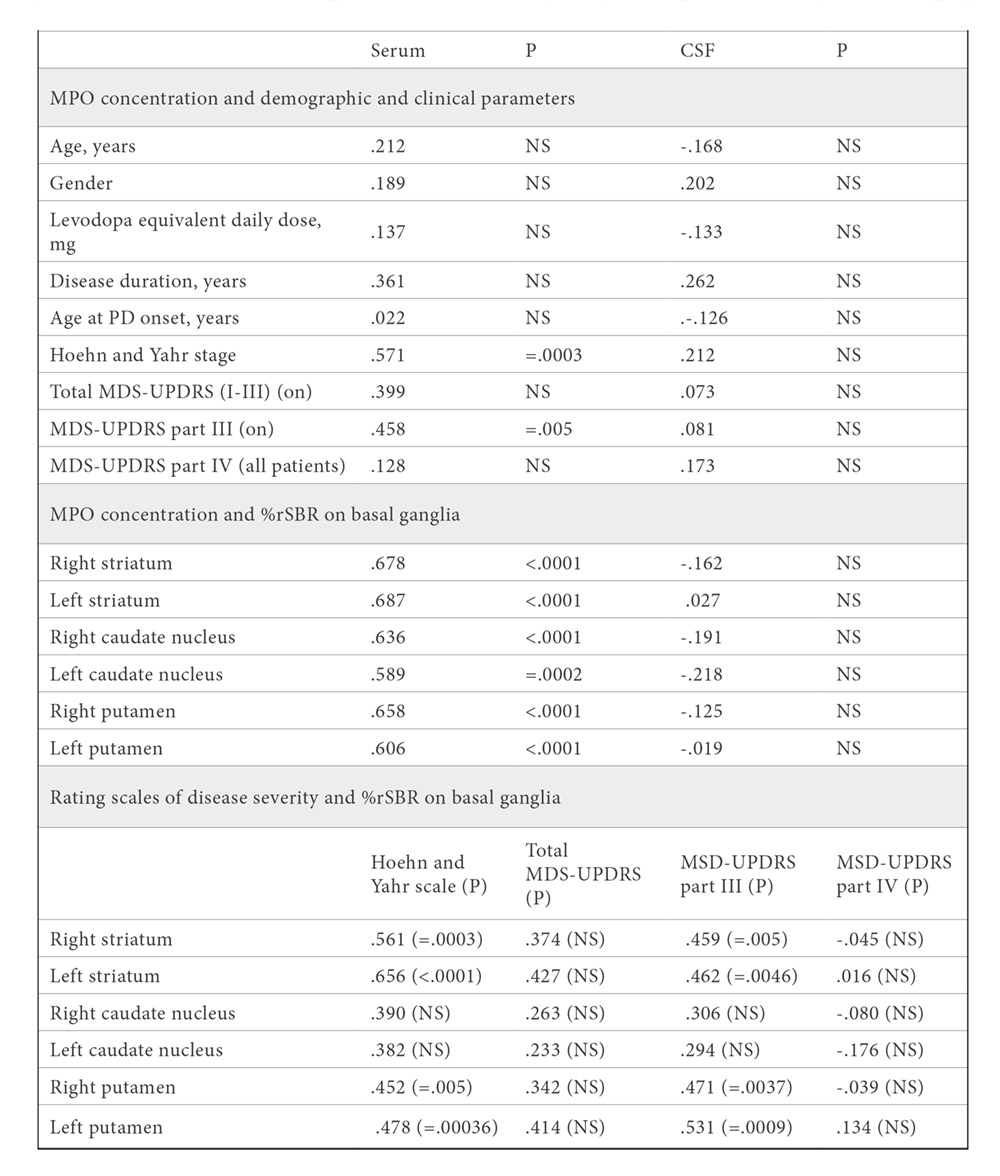
Mean ± SD. Statistical correlation was carried out with the Pearson´s test, and Bonferroni correction. Percentage reduction of dopamine-transporter binding on basal ganglia was quantified through the specific binding ratio of the radioligand 123I-Ioflupane. The size of the patients´ cohort was 36. Abbrev.: PD, Parkinson’s disease; MPO, myeloperoxidase; NS, no significant; CSF, cerebrospinal fluid; DAT-SPECT, Dopamine-transporter Single Photon Emission Computed Tomography; MDS-UPDRS, International Parkinson and Movement Disorder Society-Sponsored revision of the Unified Parkinson’s Disease Rating Scale; P, two-tailed probability value; %rSBR, percentage reduction of specific binding ratio.
Regarding DAT-SPECT data, percentage reduction of DAT binding on the striatum and putamen positively correlated with rating scales of motor severity (right and left striatum, and right and left putamen vs. Hoehn-Yahr scale, p<.005; vs. motor MDS-UPDRS part III, p<.005), as shown in Table 3 and Figure 3. Percentage reduction of DAT binding on the caudate nucleus did not significantly correlate with rating scales of motor severity. Taken together, these results indicate that the degree of loss of striatal dopaminergic neuronal terminals, as evaluated with DAT-SPECT, is well quantified with rating scales of motor severity that are commonly used in the clinics.
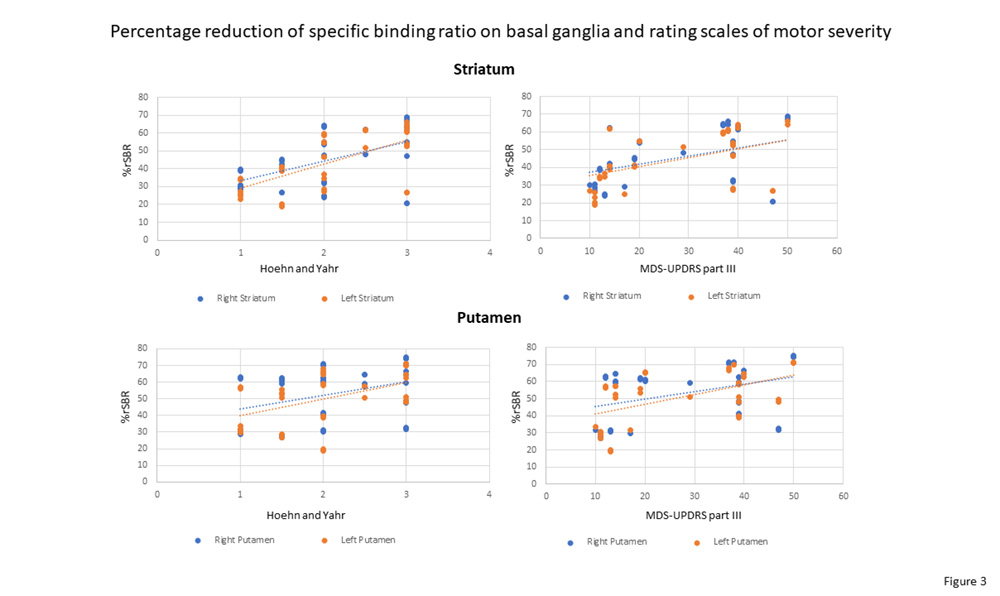
4. DISCUSSION
This study demonstrates, for the first time to the best of our knowledge, that myeloperoxidase concentration in blood serum, not in the CSF, is significantly higher in PD patients than in healthy individuals, and that serum MPO level and reduction of dopamine-transporter binding on basal ganglia are directly linked to each other. Serum MPO concentration positively correlates with degree of motor severity of PD, and patients with moderate-advanced disease (Hoehn-Yahr stage 3) present higher MPO levels than early diseased patients. The study also confirms that: a) there is a reliable reduction of dopaminergic neuronal terminals on all striatal nuclei in PD, as measured with DAT-SPECT, b) the putamen shows higher loss of DAT binding than the caudate nucleus, and c) degree of nigrostriatal dopaminergic cell loss is well quantified with rating scales of motor severity (25, 26, 30-34).
MPO has been implicated in the pathogenesis of PD. This enzyme holds promise as biomarker for diagnosis and prognosis of the disease (1-5), and it has been proposed to be a target for antiparkinsonian treatment (10, 22, 23). According to our findings, serum MPO could be a promising biomarker for diagnosis of PD, although larger studies are required to confirm it. The involvement of serum MPO in diseased patients, rather than CSF MPO, points to the implication of MPO-positive blood-derived cells, rather than brain microglia, in the pathogenesis of PD, in accordance with Gellhaar et al (10). On the other hand, MPO cytotoxicity suggests that inhibiting MPO would be useful for antiparkinsonian treatment. Jucaite et al (23) have used AZD3241, selective inhibitor of MPO, for treatment of PD patients in a clinic trial. These authors provide support for proof of mechanisms of AZD3241, although they recognize that longer treatment is required to know if the effects are beneficial for therapy.
Since oxidative stress and inflammation are involved in PD pathogenesis (6, 15-17), it can be deduced that MPO, a pro-oxidative and pro-inflammatory molecule, might play a role in this neurodegenerative disease. Blood phagocytes (neutrophils and monocytes/macrophages) are the main source of MPO, and they release hypochlorite and other reactive species that can damage neurons (3-6, 18-21). In this context, it is of great interest that, in other neuropathological conditions such as cerebral ischemia, peripheral phagocytes accumulate close to the damaged brain area and release substantial amount of hypochlorite that penetrates the brain parenchyma (11). The number of MPO-positive cells positively correlates with the volume of ischemic damage, and blood MPO concentration is highly related to stroke severity (35-37). MPO levels are found to be elevated in blood for three weeks after the ischemic episode, then returning to normal (36). If ischemia persisted, sustained elevation of the number of MPO-expressing phagocytes and MPO content in blood would occur (37).
Similar phenomena might take place in human PD. Although the results of epidemiological studies of the relationship between Parkinson’s disease and stroke are conflicting (38, 39), it can be raised up the hypothesis that PD onset would be associated with stroke in the substantia nigra. In this context, it is noteworthy that hypertension was observed to be more frequent in the cohort of patients relative to controls, and high blood pressure is a major risk factor for stroke (40). Following stroke, peripheral phagocytes would accumulate around the substantia nigra, and nigral dopaminergic neurons would be exposed to the deleterious effects of hypochlorite. It is worth noting that hypochlorite and dopamine react preferentially with each other at physiological concentrations, and they form toxic chloro-dopamine, melanic chlorinated precipitates, and aggregates of α-synuclein, the “hallmark” protein of PD pathogenesis (6, 7).
Likely a self-perpetuating process is triggered, more phagocytes would be recruited infiltrating the mesencephalic region, and excess of MPO-derived products would cause continuous neuron damage. Further investigation on these topics is warranted.
The study has some limitations that should be acknowledged. We are unable to infer causation, because it is a cross-sectional design, not a longitudinal study. Sample size was relativelly small, and larger studies are required. Serum MPO activity was not measured, and biochemical studies of enzymatic activity are also required to support the value of serum MPO as pathogenic factor in PD. Strengths of our study include well-characterized patients with PD, rigorous collection of data, the use of a very reliable SPECT technique, the use of Bonferroni correction to avoid making type I errors, and the analysis of two biofluids that are in close contact with brain tissue.
5. Conclusions
This study demonstrates that concentration of the enzyme myeloperoxidase in blood serum, but not in the CSF, is significantly higher in PD patients than in healthy individuals, and that serum MPO level and reduction of dopamine-transporter binding on basal ganglia, an indirect measure of dopaminergic neuronal loss in the substantia nigra, are directly linked to each other. Serum MPO concentration positively correlates with degree of motor severity of PD.
These results would allow improving diagnosis of PD, and they open new avenues for treatment. The study also confirms that: a) there is a reliable reduction of DAT binding on all striatal regions in PD, b) the putamen shows higher reduction of DAT binding than the caudate nucleus, and c) degree of nigrostriatal dopaminergic cell loss is well quantified with rating scales of motor severity.
Acknowledgements
The author is most grateful to all subjects who participated in this study. The authors thank Mara Guerra and Silvia Castellano (Universidad de Sevilla), and Ana Luisa Gavito (Instituto de Biomedicina de Málaga) for their technical assistance; Fernando Rodríguez de Fonseca (Instituto de Biomedicina de Málaga, Universidad Complutense de Madrid) for his unvaluable experimental help; Angel Rico, Guillermo Izquierdo, and Eva Cuartero for allowing the use of the facilities of Hospital Macarena and Valme; José-Manuel Garcia-Moreno, Ángel Martín de Pablos, and Fátima Damas-Hermoso (Servicios de Neurología y Cirugía, Hospital Macarena and Valme, Sevilla) for their excellent clinical work and biofluids collection; Cinta Calvo-Morón (Servicio de Medicina Nuclear, Hospital Macarena) and Ana Santurtún (Universidad de Cantabria) for DAT-SPECT studies, and Maria-Isabel Garcia-Sánchez and the Biobanco Hospitalario Macarena (National Biobank Network, Instituto de Salud Carlos III, Madrid) for her work with the storage of samples.
FUNDING
This work was supported by grants from Sociedad Andaluza de Neurología (ref. SUBAIA2015/006), and RETICS Red de Trastornos Adictivos, Instituto de Salud Carlos III (RD06/001/002, RD16/0017/0001). The funding sources had no further role in study design; in the collection, analysis, and interpretation of data; in writing of the report; and in the decision to submit the paper.
BIBLIOGRAPHY
- Galzigna L, De Iuliis A, Zanatta L. Enzymatic dopamine peroxidation in substantia nigra of human brain. Clin Chim Acta. 2000; 300: 131-138. https://doi.org/10.1016/S0009-8981(00)00313-2
- De Iuliis A, Burlina AP, Boschetto R, Zambenedetti P, Arslan P, Galzigna L. Increased dopamine peroxidation in postmortem Parkinsonian brain. Biochim Biophys Acta. 2002; 1573(1): 63-67. https://doi.org/10.1016/S0304-4165(02)00331-8
- Yap YW, Whiteman M, Cheung NS. Chlorinative stress: an under-appreciated mediator of neurodegeneration? Cell Signal. 2007; 19(2): 219-228. https://doi.org/10.1016/j.cellsig.2006.06.013
- Ray RS, Katyal A. Myeloperoxidase: Bridging the gap in neurodegeneration. Neurosci Biobehav Rev. 2016; 68: 611-620. https://doi.org/10.1016/j.neubiorev.2016.06.031
- Vlasova II. Peroxidase activity of human hemoproteins: Keeping the fire under control. Molecules. 2018; 23(10): 2561. https://doi.org/10.3390/molecules23102561
- Jeitner TM, Kalogiannis M, Krasnikov BF, Gomlin I, Peltier MR, Moran GR. Linking inflammation and Parkinson disease: Hypochlorous acid generates Parkinsonian poisons. Toxicol Sci. 2016; 151(2): 388-402. https://doi.org/10.1093/toxsci/kfw052
- Maki RA, Holzer M, Motamedchaboki K et al. Human myeloperoxidase (hMPO) is expressed in neurons in the substantia nigra in Parkinson’s disease and in the hMPO-α-synuclein-A53T mouse model, correlating with increased nitration and aggregation of α-synuclein and exacerbation of motor impairment. Free Radic Biol Med. 2019; 141: 115-140. https://doi.org/10.1016/j.freeradbiomed.2019.05.033
- Choi DK, Pennathur S, Perier C et al. Ablation of the inflammatory enzyme myeloperoxidase mitigates features of Parkinson’s disease in mice. J Neurosci. 2005; 25(28): 6594-6600. https://doi.org/10.1523/JNEUROSCI.0970-05.2005
- Soubhye J, Aldib I, Delporte C, Prévost M, Dufrasne F, Antwerpen PV. Myeloperoxidase as a target for the treatment of inflammatory syndromes: Mechanisms and structure activity relationships of inhibitors. Curr Med Chem. 2016; 23(35): 3975-4008. DOI: 10.2174/0929867323666160607111806
- Gellhaar S, Sunnemark D, Eriksson H, Olson L, Galter D. Myeloperoxidase-immunoreactive cells are significantly increased in brain areas affected by neurodegeneration in Parkinson’s and Alzheimer’s disease. Cell Tissue Res. 2017; 369(3): 445-454. https://doi.org/10.1007/s00441-017-2626-8
- Breckwoldt MO, Chen JW, Stangenberg L et al. Tracking the inflammatory response in stroke in vivo by sensing the enzyme myeloperoxidase. Proc Natl Acad Sci USA. 2008; 105(47): 18584-18589. https://doi.org/10.1073/pnas.0803945105
- Dunforb HB. Myeloperoxidase and eosinophil peroxidase: Phagocytosis and microbial killing. En: Dunforb HB, ed. Heme Peroxidases. New York: Wiley; 1999, p. 349-385.
- Arnhold J, Flemmig J. Human myeloperoxidase in innate and acquired immunity. Arch Biochem Biophys. 2010; 500(1): 92-106. https://doi.org/10.1016/j.abb.2010.04.008
- Klebanoff SJ, Kettle AJ, Rosen H, Winterbourn CC, Nauseef WM. Myeloperoxidase: a front-line defender against phagocytosed microorganisms. J Leukoc Biol. 2013; 93(2): 185-198. https://doi.org/10.1189/jlb.0712349
- Jenner P. Oxidative stress in Parkinson’s disease. Ann Neurol. 2003; 53(Suppl 3): S26-S38. https://doi.org/10.1002/ana.10483
- Michel PP, Hirsch EC, Hunot S. Understanding dopaminergic cell death pathways in Parkinson disease. Neuron. 2016; 90(4): 675-691. https://doi.org/10.1016/j.neuron.2016.03.038
- Navarro-Yepes J, Burns M, Anandhan A et al. Oxidative stress, redox signaling, and autophagy: Cell death versus survival. Antioxid Redox Signal. 2014 ; 21(1): 66-85. https://doi.org/10.1089/ars.2014.5837
- Hampton MB, Kettle AJ, Winterbourn CC. Inside the neutrophil phagosome: Oxidants, myeloperoxidase, and bacterial killing. Blood. 1998; 92(9): 3007-3017. PMID: 9787133
- Pullar JM, Winterbourn CC, Vissers MC. Loss of GSH and thiol enzymes in endothelial cells exposed to sublethal concentrations of hypochlorous acid. Am J Physiol. 1999; 277(4): H1505-1512. https://doi.org/10.1152/ajpheart.1999.277.4.H1505
- Bafort F, Parisi O, Perraudin JP, Jijakli MH. Mode of action of lactoperoxidase as related to its antimicrobial activity: a review. Enzyme Res. 2014; ID 517164. https://doi.org/10.1155/2014/517164
- Jeitner TM, Kalogiannis M, Patrick PA et al. Inflaming the diseased brain: a role for tainted melanins. Biochim Biophys Acta. 2015; 1852(5): 937-950. https://doi.org/10.1016/j.bbadis.2015.01.004
- Malle E, Furtmüller PG, Sattler W, Obinger C. Myeloperoxidase: a target for new drug development? Br J Pharmacol. 2007; 152(6): 838-854. https://doi.org/10.1038/sj.bjp.0707358
- Jucaite A, Svenningsson P, Rinne JO et al. Effect of the myeloperoxidase inhibitor AZD3241 on microglia: a PET study in Parkinson’s disease. Brain. 2015; 138(9): 2687-26700. https://doi.org/10.1093/brain/awv184
- Kang UJ, Goldman JG, Alcalay RN et al. The BioFIND study: Characteristics of a clinically typical Parkinson’s disease biomarker cohort. Mov. Disord. 2016; 31(6): 924-932. https://doi.org/10.1002/mds.26613
- Iranzo A, Santamaría J, Valldeoriola F et al. Dopamine transporter imaging deficit predicts early transition to synucleinopathy in idiopathic rapid eye movement sleep behavior disorder. Ann. Neurol. 2017; 82(3): 419-428. https://doi.org/10.1002/ana.25026
- Chahine LM, Iranzo A, Fernández-Arcos A et al; PPMI Sleep Working Group. Basic clinical features do not predict dopamine transporter binding in idiopathic REM behavior disorder. NPJ Parkinsons Dis. 2019; 5:2. https://doi.org/10.1038/s41531-018-0073-1
- Verber D, Novak D, Borovič M, Dugonik J, Flisar D. EQUIDopa: a responsive web application for the levodopa equivalent dose calculator. Comput. Methods Programs Biomed. 2020; 196: 105633. https://doi.org/10.1016/j.cmpb.2020.105633
- Hernán MA, Takkouche B, Caamaño-Isorna F, Gestal-Otero JJ. A meta-analysis of coffee drinking, cigarette smoking, and the risk of Parkinson’s disease. Ann Neurol. 2002; 52(3): 276-284. https://doi.org/10.1002/ana.10277
- Ragonese P, Salemi G, Morgante L et al. A case-control study on cigarette, alcohol, and coffee consumption preceding Parkinson’s disease. Neuroepidemiology. 2003; 22(5): 297-304. https://doi.org/10.1159/000071193
- Staffen W, Mair A, Unterrainer J, Trinka E, Ladurner G. Measuring the progression of idiopathic Parkinson’s disease with [123I] beta-CIT SPECT. J Neural Transm. 2000; 107: 543-552. https://doi.org/10.1007/s007020070077
- Marek K, Innis R, van Dyck C, Fussell B et al. [123I]beta-CIT SPECT imaging assessment of the rate of Parkinson’s disease progression. Neurology 2001; 57(11): 2089-2094. https://doi.org/10.1212/WNL.57.11.2089
- Nobili F, Naseri M, De Carli F et al. Automatic semi-quantification of [123I]FP-CIT SPECT scans in healthy volunteers using BasGan version 2: results from the ENC-DAT database. Eur J Nucl Med Mol Imaging. 2013; 40(4): 565-573. https://doi.org/10.1007/s00259-012-2304-8
- Varrone A, Dickson JC, Tossici-Bolt L et al. European multicentre database of healthy controls for [123I]FP-CIT SPECT (ENC-DAT): age-related effects, gender differences and evaluation of different methods of analysis. Eur J Nucl Med Mol Imaging. 2013; 40(2): 213-227. https://doi.org/10.1007/s00259-012-2276-8
- Simuni T, Uribe L, Cho HR et al; PPMI Investigators. Clinical and dopamine transporter imaging characteristics of non-manifest LRRK2 and GBA mutation carriers in the Parkinson’s Progression Markers Initiative (PPMI): a cross-sectional study. Lancet Neurol. 2020; 19(1): 71-80. doi: 10.1016/S1474-4422(19)30319-9. https://doi.org/10.1016/S1474-4422(19)30319-9
- Weston RM, Jones NM, Jarrott B, Callaway JK. Inflammatory cell infiltration after endothelin-1-induced cerebral ischemia: histochemical and myeloperoxidase correlation with temporal changes in brain injury. J Cereb Blood Flow Metab. 2007; 27(1): 100-114. https://doi.org/10.1038/sj.jcbfm.9600324
- Zheng GR, Chen B, Shen J et al. Serum myeloperoxidase concentrations for outcome prediction in acute intracerebral hemorrhage. Clin Chim Acta. 2018; 487: 330-336. https://doi.org/10.1016/j.cca.2018.10.026
- Dohi K, Ohtaki H, Nakamachi T et al. Gp91phox (NOX2) in classically activated microglia exacerbates traumatic brain injury. J Neuroinflammation. 2010; 7: 41. https://doi.org/10.1186/1742-2094-7-41
- Mastaglia FL, Johnsen RD, Kakulas BA. Prevalence of stroke in Parkinson’s disease: a postmortem study. Mov Disord. 2002; 17(4): 772-774. https://doi.org/10.1002/mds.10199
- Alves M, Caldeira D, Ferro JM, Ferreira JJ. Does Parkinson’s disease increase the risk of cardiovascular events?: a systematic review and meta-analysis. Eur J Neurol. 2020; 27(2): 288-296. https://doi.org/10.1111/ene.14076
- Buonacera A, Stancanelli B, Malatino L. Stroke and Hypertension: An Appraisal from Pathophysiology to Clinical Practice. Curr Vasc Pharmacol. 2019; 17(1): 72-84. https://dx.doi.org/10.2174/1570161115666171116151051
CONFLICT OF INTEREST STATEMENT
The author of this article declare that he has no conflict of interest with respect to what is expressed in this work.
Emilio Fernández Espejo
C/ Marco Polo, 5. Mairena del Aljarafe · 41927 Sevilla
Tlf.: +34 954 184 712 | E-Mail: efespejo@us.es
Año 2022 · número 139 (01) · páginas 44 a 54
Enviado: 28.03.22
Revisado: 03.04.22
Aceptado: 12.04.22